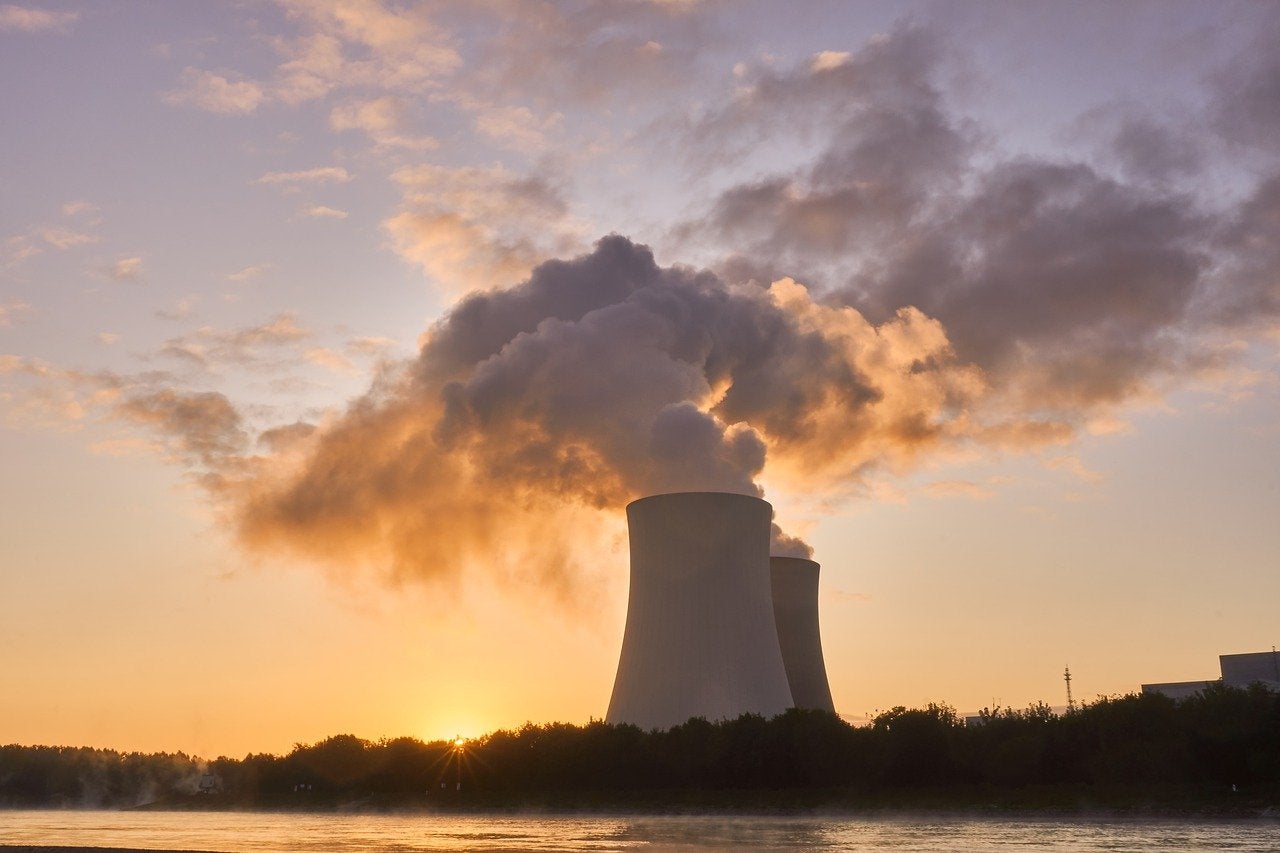
Advanced manufacturing techniques have the potential to bring great efficiency gains to the nuclear industry. They enable more complex designs, can improve the quality and safety of components, and reduce the time and cost involved in the manufacturing of components. The approaches afforded by advanced manufacturing can also strengthen supply chains against obsolescence and reduce the need for large inventories in storage. The factory-based deployment model proposed for small modular reactors (SMR) is also dependent on the introduction of several key advanced manufacturing techniques. Recently, the World Nuclear Association’s (WNA) Cooperation in Reactor Design and Licensing (CORDEL) working group produced a report based upon member feedback identifying the most promising techniques in this domain. Some of the key techniques covered by the working group are electron beam welding and hot isostatic pressing.
Electron Beam Welding (EBW)
Electron Beam Welding provides extremely strong and accurate welds without the need for a filler material. The EBW process uses local vacuum conditions and a high-power electron gun which penetrates the vessel material with an electron beam. The process melts and fuses the two components together in one go. This technique has been proven by Sheffield Forgemasters in a March 2022 demonstration and by the join Nuclear Advanced Manufacturing Research Centre (NAMRC) – Electric Power Research Institute (EPRI) project on the NuScale Power SMR reactor assemblies. These demonstrations have shown that SMR reactor vessel welds can be completed in hours rather than weeks.
Hot isostatic pressing (HIP)
Hot isostatic pressing is a manufacturing process that uses a combination of high temperature and pressure to increase the density of powdered materials into near-net shaped parts or components. This reduces the need for both machining and welding and offers the possibility of achieving complex shapes of components while also improving material properties. HIP can be used to manufacture large Class One nuclear safety components such as reactor vessel heads.
Many additive manufacturing, or 3D printing, processes exist but only a notable few are suitable for the manufacture of nuclear components. Directed energy deposition, powder bed fusion and cold spray have been identified by WNA members as the most promising. Additive manufacturing is an attractive option as it enables the production of geometric shapes that were previously impossible to make. This approach also opens the door to new possibilities for designers to improve the mechanical properties of parts while reducing their weight. Additively manufactured components have been put into service in operating nuclear power plants such as fuel debris filters, pump impellers, valve bodies and fuel channel fasteners.
While some components produced using advanced manufacturing have been placed into service, these are small items that do not have safety functions and are therefore subject to far less stringent regulations. Further development of regulations and codes and standards to support the introduction of Class One components produced via advanced manufacturing into the nuclear sector is needed.
National regulators have shown interest in the topic of advanced manufacturing and are aiming to set requirements and guidelines to review the emerging applications of the technologies to the nuclear industry. The codes and standards are used by nuclear safety authorities as a technical basis for their regulation, but they currently present gaps with regards to advanced manufacturing techniques that WNA members have identified. Some techniques are covered within codes and standards, such as electron beam welding in ASME’s Boiler & Pressure Vessel Code (BPVC). However, many gaps exist for other techniques. Examples of such gaps include a lack of specifications for raw materials, no technical specifications for products manufactured using a combination of traditional and additive manufacturing, and the absence of adequate qualification methodologies and requirements for additive manufacturing personnel.
Qualification of additive manufacturing techniques, principally laser powder bed fusion, is a notable gap as it is the most widely and commonly used technique across several industries. As such, the Nuclear Components Based on Additive Manufacturing (NUCOBAM) was launched by SNETP (Sustainable Nuclear Energy Technology Platform) in 2020. This is a project set up to develop a qualification methodology for additively manufactured components and to which several WNA members contribute. At the end of the project, NUCOBAM plans to submit the completed and final version of the qualification methodology to nuclear code and standards committees and working groups with the aim of incorporating the qualification methodology into nuclear codes and standards. Approaching the challenge with this goal and aligning the qualification with requirements from existing Design and Construction Rules for Mechanical Components of PWR Nuclear Islands (RCC-M) and ASME BPVC Section III codes should ensure that approaches to qualification for advanced manufacturing are harmonised across the two codes from the start.
However, to be truly impactful, industry initiatives such as NUCOBAM must be welcomed by standard developing organisations (SDOs) too. Approaches such as that proposed by French Association for Design, Construction and In-Service Inspection Rules for Nuclear Steam Supply System Components (AFCEN), the RCC-M code developer – which has allowed the specific use of a manufacturing process not currently referenced in the code as long as it is supported by specific documentation – will be essential. The feedback and experience then gathered from the use of the techniques will enable the introduction of technical specifications into the code for the advanced manufacturing processes. This pathway to codification will enable the processes to be introduced more quickly and let the nuclear industry benefit sooner from the benefits that advanced manufacturing offers.
The challenges of exporting reactor designs to new markets while minimising design changes due to differences in national regulatory requirements are well known and features in the WNA CORDEL “Different Interpretations of Regulatory Requirements” report. These challenges are magnified when it comes to SMRs, however, as one of their main value propositions is factory-based manufacturing in which identical module after the identical module is produced for export. Such an approach will only be possible if the standards to which they are manufactured are aligned.
The combination of growing interest in SMRs and gaps in codes and standards for advanced manufacturing, therefore, present a rare opportunity for the nuclear industry to harmonise before the technologies are commercialised. CORDEL makes a number of recommendations that actions should be taken to fully capitalise on this opportunity.
Organisations engaged in reactor design and reactor materials research should therefore dedicate more resources to the development of codes and standards as it is their staff that contribute to code development committees. Such organisations should also look to contribute to collaborative international projects that develop harmonised submissions to SDOs. Finally, SDOs should adopt a similar approach to AFCEN to enable their codes to use unreferenced advanced manufacturing processes supported by specified documentation.
This article first appeared in Nuclear Engineering International magazine.