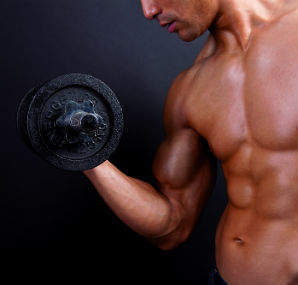
Blades are crucial components of the wind turbine, not just because they are the parts that harvest the wind’s kinetic energy, but also because the failure of a single blade can result in operational stoppages and the malfunction of the entire turbine.
Stakeholders in wind turbine projects often require certification to prove that their product is a safe and viable investment. However, despite their sleek, simple appearance, blades are complex structures, and, to the uninitiated, the certification process is a lot more complex than one might initially think. A blade has complicated geometry, material configuration and loading conditions, and, as demand for energy grows, they are becoming longer, which, in turn, increases the challenges for certification.
Wind turbines are designed and manufactured according to global standards and guidelines, such as those published by the International Electrotechnical Commission (IEC) and organisations such as classification body DNV GL. While not mandatory, they are increasingly being adopted throughout the wind industry. For example, certification is conducted in accordance with the IEC 61400 – with blades due to come under subclass IEC 61400-5 (when it is released) – to make sure they are designed and built to withstand damage from any hazards that may occur during their lifetime.
This standard provides technical conditions that are verifiable by an independent third party, such as DNV GL, and are necessary for projects to be financed and erected. The IEC 61400 covers the general aspects of turbine design, so, over the past few years, DNV GL has independently developed supplementary standards and guidelines: DNV-DS-J102 and the GL Guideline for Certification of Wind Turbines.
"We follow the IEC standard, however, in terms of the blade, it is not specific enough, so we use the DS-J102 as well as GL guidelines to check particular aspects such as safety measures, and that they are applied for strength calculations and stability," explains Tomasz Sieradzan, engineer at DNV GL.
"Blade design is changing all the time, so there needs to be flexibility within the standard, which, for the time being, the IEC does not offer. By using the IEC standard along with our own guidelines, we can provide a high-quality certificate for those that require it."
Strength in collaboration
DNV GL was established in 2013, when Norwegian organisation Det Norske Veritas (DNV) merged with Germany-based Germanischer Lloyd (GL) to become DNV GL. Both firms have a long track record in certification in markets such as offshore and shipping, as well as wind turbines, and, together, they are now the world’s largest classification society and one of the leading certification bodies for renewable energy.
"We have a very good reputation globally as a certification company," says Sieradzan. "Not only does the certification give assurance that the turbine has been checked by an independent body that has experience in the field, but it also provides security to investors and banks, and adds value to projects."
The blade-certification process starts at the design stage of a wind turbine project, usually when an investor requires verification that a project is sound or when a manufacturer wants to try out a new concept. It’s also a process of collaboration, as Sieradzan explains.
"We like to keep our clients close throughout the certification and testing process," he says. "As well as the initial technical discussions, we also explain what the testing process entails so that the customer knows what to expect.
"This includes any doubts they may have about their project, and, if the designers or manufacturers want to use a different concept, we become involved with that. I don’t think we’ve ever had a situation where we didn’t find a common agreement."
Step one: design evaluation
After the initial discussions with the client, and its designers and engineers, DNV GL checks that what the customer has on paper – materials, technical input, analyses, methods of analyses and results – is sound and follows best practice.
"This also covers looking for shortcuts in their assumptions and if there are any differences in the expected responses of the blade," says Sieradzan. "We examine the structural integrity – the strength and stability – how it behaves under certain conditions and that it fulfils conditions provided by the customer."
Blades are made of different materials, such as fibreglass and carbon fibre. Each has its own characteristics, and so the various interactions between these materials – laminate strength, differences within the carbon fibre, interfaces for the sandwich construction and interfibre failure – must be analysed and tested to see if there are any instabilities in the structure.
"It’s important to focus on the design’s details – for blade design, the devil is in the detail," adds Sieradzan. "We have to check the areas of the blade that we think are likely to cause problems, that mitigating actions have been done in the proper way, that redundancy has been incorporated and that no mistakes have been made, because even the tiniest error can come up later and have a big impact."
Step two: evaluation of manufacturing
The manufacturing evaluation is the interface check between the design and the real-life product, conducted to ensure the manufactured blade meets design specifications and that the quality control/assurance has been carried out to the highest standards.
"We check the design requirements and compare them with the standards, and then we make sure that all the critical items that contribute to the blade’s behaviour have been facilitated," explains Sieradzan. "We also look to see if the manufacturers are able to adjust the process, if necessary, in order to make the blade more robust."
Blades are huge elements – the average length is 60m, although 80m blades are in production – and are handmade with moulds. If a blade has any defects, it is usually down to human error in the manufacturing process, so, as well as looking at the blade in its entirety, areas as small as 10×10cm are scrutinised for the smallest defects.
"The blades are handmade, so examining them is tricky, because we are looking for tiny fissures and cracks, and checking that what has being done by the manufacturer actually corresponds with the design features," says Sieradzan.
At this stage, Sieradzan’s team compares the robustness of the design with the quality of the manufacturing, which includes checking the processes, materials and blade components, such as prefabricated elements for the main root.
Step three: type testing
Type-testing for blades is a sophisticated process, with each new blade or product type undergoing a lifetime (20 years) of operations through static and fatigue tests.
Static tests to verify that the blade can handle extreme loads are carried out first. This involves placing weighted loads and winches along the entire length of the blade to simulate extreme operating conditions, measuring deflection and strain, with factors applied to the test loads to compensate for any variations from the manufacturer.
During fatigue testing, loads are applied to the blade to simulate operational wear and tear, accounting for the blade’s movements when in operation – in flapwise (wind-excited) and edgewise (gravity-excited) directions, including its ability to cope with extreme loads, even on its last working day. Then, after the fatigue test, a post-fatigue static test is performed in similar conditions as the initial static test.
"Because they are handmade, every blade is a bit different," says Sieradzan. "Therefore, the testing has to consider variations in its properties. Safety load factors in the design take into account all the variations in the process, and concerns static and fatigue testing.
"Since blades are unique in their construction, with many materials involved in their structure, it’s not possible to test just the materials and get an overview of the blade; all interactions need to be checked as well."
Challenges ahead
As interest in wind energy increases – and designers develop larger yet lighter structures – so grow the challenges for certification inspectors.
"The general trend is that blades are getting longer – one manufacturer recently produced a blade of more than 80m, and there is talk about reaching lengths of up to 100m," says Sieradzan. "Five years ago, the longest one measured around 60m, so that’s a 33% increase to 80m. As blades get longer, structural challenges that were not seen before will show up."
Another growing trend is the automation of process control, which, from a certification perspective, means more interaction between design and manufacturing will be required.
"For example, in the design evaluation, we take the input for manufacturing stage and then go to manufacturing to see if all the items in the design stage are being implemented," explains Sieradzan. "This will become more pronounced in the future, especially as designers develop new concepts.
"Because we will be looking at something that is being done for the first time, we will have nothing to compare it with, so more time will be spent checking and verifying that what has been put on paper is being sufficiently implemented in production."
Wind will play a vital role in the future of renewable energy. As demand increases, and longer and more effective blades are developed, certification will be a necessary step to success.
"Our testing and certification gives assurance to stakeholders that the blades are solid, that they will operate safely and efficiently for their lifetime, and that the turbine is a safe investment," says Sieradzan.
"For as long as the blades hold, then the turbine will operate. Investors need to know that their project is free from risk, and certification from an independent party such as ourselves provides that assurance."