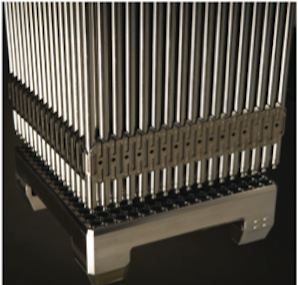
Since the 1950s, the nuclear energy industry has undergone continual, even if incremental, innovation and development. One of the greatest areas of research is nuclear fuel, especially fuel for the light water reactor (LWR) classes of boiling water reactors (BWRs) and pressurised water reactors (PWRs). From the start, BWR and PWR fuel technologies are designed with a negative moderator temperature coefficient to assure a self- limiting behaviour during power excursion events. In this frame, BWR and PWR fuel has evolved over time, both in the materials that it is made of and how it is placed inside the core of the reactor, and optimizations have been pursued that enhance fuel performance and reliability. Although the packaging of BWRs and PWRs are quite different, similar materials and geometry optimization techniques have been used to improve performance in both types of reactors.
Areas of fuel development
Initial materials inside the active fission region were created with a nuclear penalising composition such as stainless steel; these
have been replaced with Zirconium (Zr) alloys optimized for the component application.
BWRs used to feature a six by six fuel rod array; over the years, clever engineering has increased the amount of fuel reactors
can handle in a step-wise fashion, moving to a seven by seven array, then an eight by eight, nine by nine, ten by ten, and now an 11×11. Higher-order rod arrays like AREVA NP’s ATRIUMTM 11 fuel, as compared to the original six by six and seven by seven designs, have dramatically increased total length of rod within an assembly, leading to more rod surface area which, in turn, reduces surface heat flux and improves coolant mixing.
Smaller rod sizes also improve heat transfer rates and lower rod temperatures. In fact, there is a step-change reduction in the average power of each individual rod, and a corollary increase in the relationship between fuel pellet temperature and fuel rod power level. Arrays like ATRIUMTM 11 are more neutronically efficient by realising a more optimized distribution of water and fuel within the fuel bundle.
Non-uniform operating environments drive innovation for compensating features in assembly design and manufacturing, like axial and radial zoning in the lattice cross section for better fuel economics, or automated rod and assembly loading, which facilitates manufacturing. For example, BWR fuel designs have evolved to improve uranium efficiency and minimise batch sizes. Improvements in linear heat generation rate (LGHR), minimum critical power ratio (MCPR) and cold shutdown margin (CSDM) capabilities are used by the nuclear designer for more aggressive and efficient cycle designs.
Fuel channels are another essential area of improvement. These channels force the steam generated inside the fuel bundle to travel in only one direction as necessary for stable power conditions. Zirconium alloys are used to avoid parasitic neutron absorption and resist corrosion. Today’s advanced fuel channel designs minimise the total amount of metal on the exterior of the channel, so as to displace less water and improve BWR fuel economics. Machining away 25mm of a 100mm standard channel wall improves enrichment utilization by ~0.02 wt% 235U.
For PWRs, various specific optimized chemical composition, processing steps and heat treatments were implemented to obtain advanced cladding. Furthermore, advancement in pellet materials together with the advanced claddings brought a key improvement in the fuel design leading to a better fuel cycle economy. The guide tubes – which form the backbone of the fuel assembly by connecting the lower and upper end-pieces – were likewise optimized. Over the years, design adaptations have allowed engineers to increase uranium loading and double the fuel cycle length from 12 months to 24 months.
The use of spacer grids has also changed over time. These components of the fuel assembly originally provided only separation between rods and coolability after a seismic event. The span between the rods was set to minimise the risk of rod bowing, which would cause rod-to-rod contact, and to resist seismic impacts. The current generation of spacer grids has been designed to enhance fuel rod thermal performance and minimise debris entrapment risks.
Accident tolerant fuels research
In addition to these step-wise advancements, operators explore ways to incorporate additional passive safety features into the fuel itself, simply by adding or changing various materials used in the fuel’s creation. These new fuel concepts are expected to be an integral part of the future generations of fuel design for current and next-generation reactors. Their development is part of an international initiative, known as the Enhanced Accident Tolerant Fuel (EATF) programme.
To complement the initiative, the US Department of Energy (DOE) currently sponsors a programme that pulls together industry- based teams to explore different fuel design options. After the Fukushima accident, DOE recognised the need to evaluate LWR fuels and develop EATF designs. Starting in 2012, the DOE sponsored three industry-based teams to explore different fuel design options. For these designs, the DOE requires that EATF fuels:
- will give nuclear power plant operators more "coping time" to manage an accident situation;
- are deployable on or before 2022:
- continue to use the Uranium fuel cycle, with no impact; and
- are commercially feasible.
Some of the technologies being pursued include coatings on Zirconium cladding, development of alternative claddings such as Silicon Carbide (SiC) and Molybdenum-based cladding, and additives to uranium pellets. Three teams, comprising several leading US laboratories, university researchers and utility companies, were formed by AREVA NP, Westinghouse and General Electric to explore these designs. In 2016, DOE is expected to prioritise between industry leaders’ concepts and ideas that have been developed. The selected EATF designs are scheduled for deployment as lead test assemblies (LTAs) or rods in a commercial power reactor by 2022.
AREVA NP’s work with DOE’s EATF programme
AREVA NP has been at the forefront of nuclear fuel development since the invention of LWR fuel and has designed a robust investigation to support the DOE’s EATF programme. Aligned with the strict requirements to achieve LTA readiness in 2022, AREVA NP has proposed a tiered system that can deploy Zirconium alloy fuel rods coated with Chromium (Cr) in LTAs in advance of the 2022 deadline.
Chromium coated Zirconium alloy cladding, developed by the CEA within the French Nuclear Institute (AREVA, CEA and EDF),
and achieves added protection against high temperature oxidation in a severe accident. The protective Chromium coating gives
control room operators additional time to return the plant to normal or shut down conditions before the onset of fuel damage. When paired with AREVA NP’s Cr2O3 pellet design, these fuel rods can retain fission gas better than standard UO2 and may provide additional steam and water resistance in the event of a fuel rod rupture. AREVA NP has also embarked on SiC/SiC composite cladding development within the French Nuclear Institute to further enhance accident tolerance.
Separately, the Electric Power Research Institute (EPRI) has partnered with AREVA Federal Services, a subsidiary of the US-based AREVA Inc, to develop a Molybdenum cladding that can resist extreme temperatures and especially maintain coolable geometry in accident conditions.
Through its partnership with the University of Florida, AREVA NP is also exploring the use of advanced manufacturing techniques known as Spark Plasma Sintering (SPS) that offer economic advantages by significantly reducing power consumption and per pellet
processing time. These advanced concepts have the potential to further increase the reactor operator coping time and potentially prevent fuel rod damage in a severe accident and increase value to utilities. Pellets loaded with SiC or diamond additives can exhibit increased thermal conductivity. AREVA NP has initiated irradiation programmes in the Idaho National Lab’s Advanced Test Reactor to begin detailed characterisation of these modified pellets, with the first results expected in late 2016.
In addition to leading one of DOE’s EATF exploration teams, AREVA NP is also involved with an EATF project with the Gösgen Nuclear Power Plant in Switzerland. We will supply test rods containing innovative cladding materials. Developed by CEA in partnership with AREVA and EDF, these materials will be regularly examined in PSI’s2 and CEA’s hot cells. Based on the confirmation of expected gains, the best solutions will be commercialized as of 2020.
Ongoing implementation of advanced fuel
Our engineers are also currently deploying new fuel designs – ATRIUMTM 11 in the BWR market and GAIA in the PWR market.
ATRIUMTM 11 BWR fuel design features a symmetric 11×11 array of 112 fuel rods to achieve the required fuel cycle cost, reliability and operational flexibility improvements. Fuel rods are loaded with Cr2O3 doped fuel pellets. Testing of BWR fuel has demonstrated that Cr2O3 pellets provide enhanced protection against pellet-clad interaction failure (PCI) comparable to that brought by liner cladding. Considering that the non-liner part of the cladding provides the primary mechanical structure (ie, the structural wall), combining Cr2O3 doped fuel with non-liner cladding it results in a greater absolute thickness of the cladding structural wall relative to a ten by ten fuel rod with liner cladding, making available the potential for increased mechanical margin.
It is designed to deliver a fuel efficiency gain two to three percent beyond current ten by ten products. By moving to an 11×11 array, the average linear heat generation rate (LHGR) is reduced by 19%. This has benefits for operating flexibility that can lead to further economic gains by increasing plant capacity factors.
The design features are complemented by the use of our Z4BTM alloy for the external fuel channel structure. Z4BTM is a derivative of Zry-4 with optimized iron and chromium levels to improve corrosion resistance. The external fuel channel material receives a beta- quench heat treatment in the final thickness to produce a randomized microstructure. The combination of Z4BTM and beta-quenching effectively eliminates fluence-induced growth to improve dimensional stability and minimise risk of control blade interference.
Several elements of the ATRIUMTM 11 fuel assembly contribute to its comprehensive defence against debris fretting. A third generation FUELGUARDTM inlet debris filter increases operational reliability by preventing all debris large enough to damage the fuel rod array from entering the assembly with the reactor coolant; a closed grid upper tie plate protects the fuel rod array from debris entering from above, for example during refuelling operations. Assuming that smallest debris may eventually be introduced into the fuel rod array, the ATRIUMTM 11 features a low entrapment spacer grid that works to move debris away from the fuel rod contact points such that it can safely pass through the open areas between the fuel rods.
Right now, the design is being irradiated in lead quantities in three European reactors and in two in the US. In total there are currently
40 assemblies that have been operating without incident worldwide since 2012. A comprehensive inspection programme will follow and a number of post-irradiation examinations have been completed showing expected performance. Based on the worldwide experience, initial ATRIUMTM 11 reload supplies are expected in 2018.
Meanwhile, our GAIA fuel rod design uses M5TM loaded with chamfered pellets doped with Cr2O3. The result is state-of-the- art performance with low corrosion, high density, low fission release and excellent PCI resistance. Numerous tests on the GAIA grid have confirmed the excellent grid to rod fretting resistance at the level of HTPTM. AUTOCLAVE testing under simulated end- of-life conditions has been performed for 1,500 hours. Additional endurance tests have been performed on full-scale bundle in the HERMES-P loop located at CEA Cadarache, a French scientific research centre that specialises in nuclear power research. These tests were conducted in PWR hydraulic conditions and further confirm the low wear at the grid to cladding interface. GAIA mixing grids combine state-of-the-art grid to rod fretting and thermal hydraulic performance. Further, the outer grid strap design is optimized to prevent inter-assembly hang-up to offer improved fuel handling. Design optimisations of the GAIA grid have allowed achieving a performance level above the AFA 3GTM design. The addition of intermediate mixing grids in the upper sections of the fuel assemblies further increases thermal hydraulic performance to satisfy the needs of the most demanding reactors.
The MONOBLOCTM guide tubes are fabricated with the new quaternary alloy of zirconium Q12TM to significantly increase resistance to lateral assembly deformation. Finally, the lower nozzle has been redesigned to simultaneously trap all PWR debris forms while reducing pressure drop and offering additional vibration resistance through seating the fuel rods directly in the nozzle.
Currently, the GAIA design is being irradiated in lead quantities in Europe and in the US. The associated extensive Post Irradiation Examination (PIE) programme continues to demonstrate GAIA performance to the requirements established to meet market needs.
Next steps
Within the next few years, the industry will begin to see the impact of today’s extensive R&D programmes on nuclear fuels. While advanced fuel designs are already available, future generation fuels and EATF fuels represent the next phase of the nuclear industry’s growth. Fuels will continue to advance, especially as new reactor designs like small nuclear reactors, molten salt reactors, pebble bed reactors and travelling wave reactors begin to be constructed around the globe.
Author Bios
John Strumpell is manager of US Fuel Research and Development at AREVA NP in Lynchburg, Va. He has been with AREVA since 2008. Previously he worked as a design engineer for Texas Instruments. Strumpell holds a Master of Science in mechanical engineering from Southern Methodist University and a Bachelor of Science in mechanical engineering from Louisiana Tech University.
Steve Cole is a product line manager at AREVA NP’s fuel facility in Richland, Washington. He began working with AREVA’s Fuel Business in 2000. Previously he was a nuclear engineer for Siemens. Cole holds an MBA from Washington State University and a Bachelor of Science in nuclear engineering from Oregon State University.
Pierre Mollard, based in Lyon, France, is business-to-business and customer relations manager for the Contracts and Services division of AREVA NP’s Fuel Business Line. Pierre has been with AREVA since 1988. He is a nuclear engineer, having received his degree from the École Nationale Supérieure d’Ingénieurs Électriciens de Grenoble (ENSIEG) in Grenoble, France.
References
[1] Commissariat à l’Energie Atomique et aux Energies Alternatives (www.cea.fr)
[2] Paul Scherrer Institut (www.psi.ch)